Welcome
to Our Site
Welcome visitors to your site with a short, engaging introduction. Double click to edit and add your own text.
Quantum Mechanical Description of the Atom and Its Electronic Structure
Introduction to Quantum Mechanics
-
Wave-Particle Duality: Electrons exhibit both particle and wave characteristics, which is a fundamental concept in quantum mechanics. This duality suggests that the behavior of electrons can change depending on the experimental context, leading to phenomena that classical physics struggles to explain. For instance, electrons can produce interference patterns like waves in the double-slit experiment [2][6].
-
Heisenberg Uncertainty Principle: This principle asserts that it is impossible to precisely determine both the position and momentum of an electron at the same time. The more accurately one of these quantities is known, the less precisely the other can be determined. This uncertainty is inherent in the nature of quantum systems [1][4].
-
Schrödinger Equation: This equation plays a central role in quantum mechanics by describing the wave function of a system. It allows for the calculation of probabilities regarding an electron’s location and momentum, providing insights into the behavior of electrons in atoms [1][3].
Atomic Orbitals
-
Types of Orbitals:
-
s Orbitals: Spherical in shape; can hold 2 electrons.
-
p Orbitals: Dumbbell-shaped; can hold 6 electrons (3 p-orbitals per energy level).
-
d Orbitals: Complex shapes; can hold 10 electrons (5 d-orbitals per energy level).
-
f Orbitals: Even more complex; can hold 14 electrons (7 f-orbitals per energy level) [3][7].
-
Quantum Numbers
Quantum numbers describe the properties of atomic orbitals and the electrons within them:
-
Principal (n): Indicates the main energy level or shell.
-
Azimuthal (l): Describes the shape of the orbital (0 = s, 1 = p, 2 = d, 3 = f).
-
Magnetic (m_l): Describes the orientation of the orbital.
-
Spin (m_s): Describes the direction of electron spin (±1/2) [3][8].
Electron Configuration
Principles Governing Electron Configuration:
-
Aufbau Principle: Electrons fill the lowest energy orbitals first.
-
Pauli Exclusion Principle: No two electrons in an atom can have identical sets of quantum numbers.
-
Hund’s Rule: Electrons will fill degenerate orbitals singly before pairing up to minimize repulsion between them [5][8].
Example: The electron configuration for oxygen (O) is 1s22s22p41s^2 2s^2 2p^4.
Energy Levels and Orbitals
Energy levels are quantized, meaning electrons can only occupy specific energy states. The further an orbital is from the nucleus, the higher its energy [3][7].
Arrangement of Elements in the Periodic Table and Trends in Properties
Periodic Law
The periodic law states that the properties of elements exhibit periodic repetition when arranged in increasing atomic number. This periodicity is due to the recurring patterns in electron configurations [3][7].
Periodic Trends
-
Atomic Radius:
-
Decreases across a period due to increased nuclear charge pulling electrons closer to the nucleus.
-
Increases down a group due to additional electron shells, which shield the outer electrons from the nucleus.
-
-
Ionization Energy:
-
The energy required to remove an electron from an atom. It increases across a period and decreases down a group as atomic size increases.
-
-
Electron Affinity:
-
The energy change when an electron is added to an atom. It becomes more negative across a period as atoms become more capable of attracting electrons.
-
-
Electronegativity:
-
A measure of an atom's ability to attract electrons in a bond. It increases across a period and decreases down a group [6][8].
-
Block Classification
-
S-block: Groups 1 and 2 (includes hydrogen and helium).
-
P-block: Groups 13–18.
-
D-block: Transition metals (Groups 3–12).
-
F-block: Lanthanides and actinides [6][8].
Ionic Bond Formation in Terms of Atomic Properties
Ionic Bonding Basics
Ionic bonds are formed when one atom transfers one or more electrons to another atom, resulting in the creation of ions—cations (positively charged) from metals and anions (negatively charged) from nonmetals [8].
Electron Transfer
-
Metals like sodium (Na) lose electrons to achieve a stable electron configuration, forming cations.
-
Nonmetals like chlorine (Cl) gain electrons to form anions [7][8].
Atomic Properties Involved
-
Ionization Energy: Metals typically have low ionization energies, making it easier for them to lose electrons.
-
Electron Affinity: Nonmetals tend to have high electron affinities, which makes them more likely to gain electrons.
-
Electronegativity: A significant difference in electronegativity between two atoms increases the likelihood of ionic bond formation.
Example: In sodium chloride (NaCl), sodium loses one electron to become Na⁺, while chlorine gains one electron to become Cl⁻. The oppositely charged ions attract each other, resulting in the formation of an ionic bond due to electrostatic attraction [7][8].
Citations:
[1] https://www.energy.gov/science/doe-explainsquantum-mechanics
[2] https://en.wikipedia.org/wiki/Wave_particle_duality
[3] https://chem.libretexts.org/Courses/College_of_the_Canyons/Chem_201:_General_Chemistry_I_OER/09:_Electronic_Structure_and_Periodic_Table/9.04:_The_Quantum-Mechanical_Model_of_an_Atom
[4] https://chem.libretexts.org/Courses/University_of_Arkansas_Little_Rock/Chem_1402:_General_Chemistry_1_(Kattoum)/Text/6:_The_Structure_of_Atoms/6.4:_Particle-Wave_Duality:_Prelude_to_Quantum_Mechanics
[5] https://www.oakparkusd.org/cms/lib5/CA01000794/Centricity/Domain/863/QuantumMechanicalModel.pdf
[6] https://www.livescience.com/wave-particle-duality
[7] https://www.jove.com/science-education/11677/electronic-structure-of-atoms-quantum-mechanical-model
[8] https://ecampusontario.pressbooks.pub/enhancedchemistry/chapter/quantum-mechanical-atom/
Properties of Ionic Compounds in Relation to Their Structure
Crystal Lattice Structure
Ionic compounds are characterized by a crystal lattice structure, a three-dimensional arrangement of ions held together by strong electrostatic forces. This regular, repeating pattern leads to a stable configuration that minimizes potential energy.
Properties of Ionic Compounds
-
High Melting and Boiling Points: The strong electrostatic forces between oppositely charged ions require substantial energy to overcome, resulting in high melting and boiling points. For example, sodium chloride (NaCl) has a melting point of about 801°C [2][3].
-
Electrical Conductivity: Ionic compounds can conduct electricity when melted or dissolved in water, as the ions are free to move. In solid form, the ions are fixed in place within the lattice and cannot move to conduct electricity [2][3][7].
-
Solubility in Water: Many ionic compounds dissolve in water because the polar water molecules can surround and separate the ions, overcoming the attractive forces holding them together in the lattice structure [1][4].
-
Hard and Brittle: Ionic compounds are typically hard due to their rigid structure. However, they are brittle; when enough force is applied, like charges may align, repelling each other and causing the crystal to shatter [1][2].
Example: Sodium chloride (NaCl) forms a cubic lattice where each Na⁺ ion is surrounded by six Cl⁻ ions and vice versa, illustrating the orderly arrangement typical of ionic compounds.
Covalent Bond Formation in Terms of Atomic Properties
Covalent Bonding Basics
Covalent bonds form when two nonmetals share electrons, allowing both atoms to achieve stable electron configurations, often resembling those of noble gases.
Atomic Properties Involved
-
Electronegativity: In covalent bonds, atoms with similar electronegativities share electrons equally. If there is a significant difference in electronegativity, the bond becomes polar, creating partial positive and negative charges on the atoms.
-
Bond Length and Bond Strength: The strength of a bond is inversely related to its length—shorter bonds (like triple bonds) are stronger than longer bonds (like single bonds). For example, a double bond is stronger than a single bond but weaker than a triple bond.
Lewis Structures and Bond Formation
Lewis structures visually represent electrons as dots around atoms, with shared pairs depicted as lines, indicating covalent bonds.
Example: In a hydrogen molecule (H₂), two hydrogen atoms share a pair of electrons, forming a single covalent bond.
Properties of Molecular Covalent Compounds in Relation to Their Structure
Molecular Structure
According to VSEPR Theory (Valence Shell Electron Pair Repulsion), molecules adopt shapes that minimize electron pair repulsion, leading to specific molecular geometries.
Common Geometries
-
Linear
-
Bent
-
Trigonal planar
-
Tetrahedral
-
Trigonal bipyramidal
-
Octahedral
Properties of Molecular Compounds
-
Low Melting and Boiling Points: Covalent bonds within molecules are generally weaker than ionic bonds, resulting in lower melting and boiling points compared to ionic compounds.
-
Non-conductivity: Molecular
compounds do not conduct electricity because they lack free-moving ions or electrons.
-
Solubility: Molecular compounds tend to dissolve in nonpolar solvents but may be insoluble in polar solvents like water.
Example: Water (H₂O) has a bent molecular shape and is polar, contributing to its relatively high boiling point compared to nonpolar molecules like nitrogen (N₂).
The Properties of Organic Compounds and Polymers in Terms of Their Structure
Organic Compounds
-
Hydrocarbons: Composed solely of hydrogen and carbon; they are categorized into alkanes (single bonds), alkenes (double bonds), and alkynes (triple bonds).
-
Functional Groups: Specific atom groupings that define chemical reactivity, such as alcohols (–OH), carboxylic acids (–COOH), and amines (–NH₂).
-
Isomerism: Organic compounds can have the same molecular formula but differ structurally (structural isomers) or spatially (stereoisomers).
Polymers
-
Addition Polymers: Formed by the addition of monomers with double bonds (e.g., polyethylene).
-
Condensation Polymers: Created by eliminating small molecules like water during polymerization (e.g., nylon, polyester).
Structure-Property Relationships in Polymers
-
Chain Length: Longer chains generally result in stronger materials.
-
Branching: Increased branching can reduce density and increase flexibility.
-
Crosslinking: Crosslinked polymers form a three-dimensional network, enhancing strength and rigidity (e.g., rubber).
Example: Polyethylene, derived from ethene (C₂H₄), exhibits varying properties depending on the polymerization degree and whether it is branched or linear.
These properties highlight how structure influences the behavior of different types of compounds, from ionic solids to organic polymers.
Citations:
[1] https://socratic.org/questions/the-crystal-lattice-structure-of-ionic-compounds-is-responsible-for-which-set-of
[2] https://study.com/academy/lesson/ionic-compounds-formation-lattice-energy-and-properties.html
[3] https://chem.libretexts.org/Courses/Bellarmine_University/BU:_Chem_103_(Christianson)/Phase_3:_Atoms_and_Molecules_-_the_Underlying_Reality/9:_Chemical_Bonding/9.2:_Ionic_Bonding_and_Lattice_Energy
[4] https://www.bbc.co.uk/bitesize/guides/ztc6w6f/revision/3
[5] https://people.wou.edu/~courtna/ch412/lattice.html
[6] https://www.tf.uni-kiel.de/matwis/amat/def_en/kap_2/basics/b2_1_6.html
[7] https://en.wikipedia.org/wiki/Ionic_crystal
[8] https://flexbooks.ck12.org/cbook/ck-12-chemistry-flexbook-2.0/section/8.7/primary/lesson/ionic-crystal-structure-chem/
Let me know if you'd like further adjustments or clarifications!
Here’s a revised version of the text with corrections for consistency, clarity, and readability:
Quantum Mechanical Description of the Atom and Its Electronic Structure
Introduction to Quantum Mechanics
-
Wave-Particle Duality: Electrons exhibit both particle and wave characteristics, which is a fundamental concept in quantum mechanics. This duality suggests that the behavior of electrons can change depending on the experimental context, leading to phenomena that classical physics struggles to explain. For instance, electrons can produce interference patterns like waves in the double-slit experiment [2][6].
-
Heisenberg Uncertainty Principle: This principle asserts that it is impossible to precisely determine both the position and momentum of an electron at the same time. The more accurately one of these quantities is known, the less precisely the other can be determined. This uncertainty is inherent in the nature of quantum systems [1][4].
-
Schrödinger Equation: This equation plays a central role in quantum mechanics by describing the wave function of a system. It allows for the calculation of probabilities regarding an electron’s location and momentum, providing insights into the behavior of electrons in atoms [1][3].
Atomic Orbitals
-
Types of Orbitals:
-
s Orbitals: Spherical in shape; can hold 2 electrons.
-
p Orbitals: Dumbbell-shaped; can hold 6 electrons (3 p-orbitals per energy level).
-
d Orbitals: Complex shapes; can hold 10 electrons (5 d-orbitals per energy level).
-
f Orbitals: Even more complex; can hold 14 electrons (7 f-orbitals per energy level) [3][7].
-
Quantum Numbers
Quantum numbers describe the properties of atomic orbitals and the electrons within them:
-
Principal (n): Indicates the main energy level or shell.
-
Azimuthal (l): Describes the shape of the orbital (0 = s, 1 = p, 2 = d, 3 = f).
-
Magnetic (m_l): Describes the orientation of the orbital.
-
Spin (m_s): Describes the direction of electron spin (±1/2) [3][8].
Electron Configuration
Principles Governing Electron Configuration:
-
Aufbau Principle: Electrons fill the lowest energy orbitals first.
-
Pauli Exclusion Principle: No two electrons in an atom can have identical sets of quantum numbers.
-
Hund’s Rule: Electrons will fill degenerate orbitals singly before pairing up to minimize repulsion between them [5][8].
Example: The electron configuration for oxygen (O) is 1s22s22p41s^2 2s^2 2p^4.
Energy Levels and Orbitals
Energy levels are quantized, meaning electrons can only occupy specific energy states. The further an orbital is from the nucleus, the higher its energy [3][7].
Arrangement of Elements in the Periodic Table and Trends in Properties
Periodic Law
The periodic law states that the properties of elements exhibit periodic repetition when arranged in increasing atomic number. This periodicity is due to the recurring patterns in electron configurations [3][7].
Periodic Trends
-
Atomic Radius:
-
Decreases across a period due to increased nuclear charge pulling electrons closer to the nucleus.
-
Increases down a group due to additional electron shells, which shield the outer electrons from the nucleus.
-
-
Ionization Energy:
-
The energy required to remove an electron from an atom. It increases across a period and decreases down a group as atomic size increases.
-
-
Electron Affinity:
-
The energy change when an electron is added to an atom. It becomes more negative across a period as atoms become more capable of attracting electrons.
-
-
Electronegativity:
-
A measure of an atom's ability to attract electrons in a bond. It increases across a period and decreases down a group [6][8].
-
Block Classification
-
S-block: Groups 1 and 2 (includes hydrogen and helium).
-
P-block: Groups 13–18.
-
D-block: Transition metals (Groups 3–12).
-
F-block: Lanthanides and actinides [6][8].
Ionic Bond Formation in Terms of Atomic Properties
Ionic Bonding Basics
Ionic bonds are formed when one atom transfers one or more electrons to another atom, resulting in the creation of ions—cations (positively charged) from metals and anions (negatively charged) from nonmetals [8].
Electron Transfer
-
Metals like sodium (Na) lose electrons to achieve a stable electron configuration, forming cations.
-
Nonmetals like chlorine (Cl) gain electrons to form anions [7][8].
Atomic Properties Involved
-
Ionization Energy: Metals typically have low ionization energies, making it easier for them to lose electrons.
-
Electron Affinity: Nonmetals tend to have high electron affinities, which makes them more likely to gain electrons.
-
Electronegativity: A significant difference in electronegativity between two atoms increases the likelihood of ionic bond formation.
Example: In sodium chloride (NaCl), sodium loses one electron to become Na⁺, while chlorine gains one electron to become Cl⁻. The oppositely charged ions attract each other, resulting in the formation of an ionic bond due to electrostatic attraction [7][8].
Citations:
[1] https://www.energy.gov/science/doe-explainsquantum-mechanics
[2] https://en.wikipedia.org/wiki/Wave_particle_duality
[3] https://chem.libretexts.org/Courses/College_of_the_Canyons/Chem_201:_General_Chemistry_I_OER/09:_Electronic_Structure_and_Periodic_Table/9.04:_The_Quantum-Mechanical_Model_of_an_Atom
[4] https://chem.libretexts.org/Courses/University_of_Arkansas_Little_Rock/Chem_1402:_General_Chemistry_1_(Kattoum)/Text/6:_The_Structure_of_Atoms/6.4:_Particle-Wave_Duality:_Prelude_to_Quantum_Mechanics
[5] https://www.oakparkusd.org/cms/lib5/CA01000794/Centricity/Domain/863/QuantumMechanicalModel.pdf
[6] https://www.livescience.com/wave-particle-duality
[7] https://www.jove.com/science-education/11677/electronic-structure-of-atoms-quantum-mechanical-model
[8] https://ecampusontario.pressbooks.pub/enhancedchemistry/chapter/quantum-mechanical-atom/
Properties of Ionic Compounds in Relation to Their Structure
Crystal Lattice Structure
Ionic compounds are characterized by a crystal lattice structure, a three-dimensional arrangement of ions held together by strong electrostatic forces. This regular, repeating pattern leads to a stable configuration that minimizes potential energy.
Properties of Ionic Compounds
-
High Melting and Boiling Points: The strong electrostatic forces between oppositely charged ions require substantial energy to overcome, resulting in high melting and boiling points. For example, sodium chloride (NaCl) has a melting point of about 801°C [2][3].
-
Electrical Conductivity: Ionic compounds can conduct electricity when melted or dissolved in water, as the ions are free to move. In solid form, the ions are fixed in place within the lattice and cannot move to conduct electricity [2][3][7].
-
Solubility in Water: Many ionic compounds dissolve in water because the polar water molecules can surround and separate the ions, overcoming the attractive forces holding them together in the lattice structure [1][4].
-
Hard and Brittle: Ionic compounds are typically hard due to their rigid structure. However, they are brittle; when enough force is applied, like charges may align, repelling each other and causing the crystal to shatter [1][2].
Example: Sodium chloride (NaCl) forms a cubic lattice where each Na⁺ ion is surrounded by six Cl⁻ ions and vice versa, illustrating the orderly arrangement typical of ionic compounds.
Covalent Bond Formation in Terms of Atomic Properties
Covalent Bonding Basics
Covalent bonds form when two nonmetals share electrons, allowing both atoms to achieve stable electron configurations, often resembling those of noble gases.
Atomic Properties Involved
-
Electronegativity: In covalent bonds, atoms with similar electronegativities share electrons equally. If there is a significant difference in electronegativity, the bond becomes polar, creating partial positive and negative charges on the atoms.
-
Bond Length and Bond Strength: The strength of a bond is inversely related to its length—shorter bonds (like triple bonds) are stronger than longer bonds (like single bonds). For example, a double bond is stronger than a single bond but weaker than a triple bond.
Lewis Structures and Bond Formation
Lewis structures visually represent electrons as dots around atoms, with shared pairs depicted as lines, indicating covalent bonds.
Example: In a hydrogen molecule (H₂), two hydrogen atoms share a pair of electrons, forming a single covalent bond.
Properties of Molecular Covalent Compounds in Relation to Their Structure
Molecular Structure
According to VSEPR Theory (Valence Shell Electron Pair Repulsion), molecules adopt shapes that minimize electron pair repulsion, leading to specific molecular geometries.
Common Geometries
-
Linear
-
Bent
-
Trigonal planar
-
Tetrahedral
-
Trigonal bipyramidal
-
Octahedral
Properties of Molecular Compounds
-
Low Melting and Boiling Points: Covalent bonds within molecules are generally weaker than ionic bonds, resulting in lower melting and boiling points compared to ionic compounds.
-
Non-conductivity: Molecular
compounds do not conduct electricity because they lack free-moving ions or electrons.
-
Solubility: Molecular compounds tend to dissolve in nonpolar solvents but may be insoluble in polar solvents like water.
Example: Water (H₂O) has a bent molecular shape and is polar, contributing to its relatively high boiling point compared to nonpolar molecules like nitrogen (N₂).
The Properties of Organic Compounds and Polymers in Terms of Their Structure
Organic Compounds
-
Hydrocarbons: Composed solely of hydrogen and carbon; they are categorized into alkanes (single bonds), alkenes (double bonds), and alkynes (triple bonds).
-
Functional Groups: Specific atom groupings that define chemical reactivity, such as alcohols (–OH), carboxylic acids (–COOH), and amines (–NH₂).
-
Isomerism: Organic compounds can have the same molecular formula but differ structurally (structural isomers) or spatially (stereoisomers).
Polymers
-
Addition Polymers: Formed by the addition of monomers with double bonds (e.g., polyethylene).
-
Condensation Polymers: Created by eliminating small molecules like water during polymerization (e.g., nylon, polyester).
Structure-Property Relationships in Polymers
-
Chain Length: Longer chains generally result in stronger materials.
-
Branching: Increased branching can reduce density and increase flexibility.
-
Crosslinking: Crosslinked polymers form a three-dimensional network, enhancing strength and rigidity (e.g., rubber).
Example: Polyethylene, derived from ethene (C₂H₄), exhibits varying properties depending on the polymerization degree and whether it is branched or linear.
These properties highlight how structure influences the behavior of different types of compounds, from ionic solids to organic polymers.
Citations:
[1] https://socratic.org/questions/the-crystal-lattice-structure-of-ionic-compounds-is-responsible-for-which-set-of
[2] https://study.com/academy/lesson/ionic-compounds-formation-lattice-energy-and-properties.html
[3] https://chem.libretexts.org/Courses/Bellarmine_University/BU:_Chem_103_(Christianson)/Phase_3:_Atoms_and_Molecules_-_the_Underlying_Reality/9:_Chemical_Bonding/9.2:_Ionic_Bonding_and_Lattice_Energy
[4] https://www.bbc.co.uk/bitesize/guides/ztc6w6f/revision/3
[5] https://people.wou.edu/~courtna/ch412/lattice.html
[6] https://www.tf.uni-kiel.de/matwis/amat/def_en/kap_2/basics/b2_1_6.html
[7] https://en.wikipedia.org/wiki/Ionic_crystal
[8] https://flexbooks.ck12.org/cbook/ck-12-chemistry-flexbook-2.0/section/8.7/primary/lesson/ionic-crystal-structure-chem/
Let me know if you'd like further adjustments or clarifications!
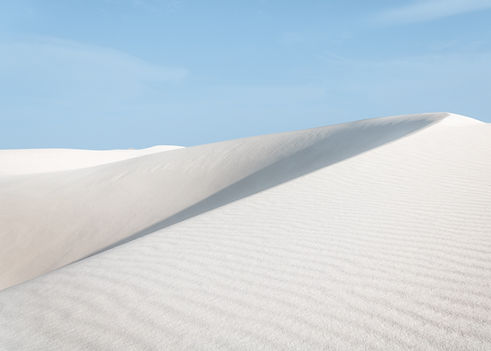